Volume 3, Year 2016 - Pages 7-10
DOI: TBD
CO2 Separation through Carbon Tubular Membranes: Effect of Polymer Composition
N. Sazali1, 2, W.N.W. Salleh1, A.F. Ismail1, N.A.H.M. Nordin1, Zawati Harun2
1Advanced Membrane Technology Research Centre (AMTEC), Faculty of Chemical and Energy Engineering,
Universiti Teknologi Malaysia, 81310 Skudai, Johor Darul Takzim, Malaysia
Melya.jandi@yahoo.com; hayati@petroleum.utm.my; afauzi@utm.my; nahadi2@live.utm.my
2Advanced Materials and Manufacturing Centre (AMMC), Faculty of Mechanical and Manufacturing Engineering
Universiti Tun Hussein Onn Malaysia, 86400 Parit Raja, Batu Pahat, Johor Darul Takzim, Malaysia
zawati@uthm.edu.my
Abstract - Carbon membrane can be regarded as the future of the separation media in gas separation process due to its high gas separation performance, ease process ability, and moderate energy requirement as compared to conventional separation. In this study, the influence of the polymer (Matrimid 5218) composition; 5wt%, 10wt%, 13wt%, 15wt%, and 18wt%, on the gas permeation properties was investigated. The supported polymer precursor was prepared by coated the polymer solution on TiO2 –ZrO2 tubes by using dip-coating method before undergoes carbonization at 850°C under Nitrogen atmosphere. Pure gas permeation tests were performed by using CH4, and CO2, at room temperature and pressure of 8 bars. It was found that polymer composition plays a significant role in the carbon membrane preparation. The highest CO2/CH4 selectivity of 83.30 was obtained for carbon membrane prepared from 15wt% polymer composition.
Keywords: Polymeric precursor, heat treatment process, Permeation, polymer concentration, carbon membrane, gas separation.
© Copyright 2016 Authors - This is an Open Access article published under the Creative Commons Attribution License terms. Unrestricted use, distribution, and reproduction in any medium are permitted, provided the original work is properly cited.
Date Received: 2014-08-09
Date Accepted: 2016-10-27
Date Published: 2016-11-09
1. Introduction
Membranes separation processes are not a recent invention, which have experienced a fast development amid the previous couple of decades. Membranes and membrane processes were initially presented as an analytical tool in chemical and biomedical laboratories: they formed quickly into industrial products and techniques with huge specialized in technical and commercial value [10]. Polymeric membranes, usually in the form of flat sheet or hollow fibres are capable of achieving moderate permeability and selectivity, with lower cost and easy manufacturing processes [11]. While these membranes have been the interest in gas separation industries [6], limitation such as performance trade-off revealed as Robeson upper-bound relationship has been one of the underlying reason for its slow growth for commercialization [10]. The inadequacies of polymeric membranes in separation process have encouraged the researchers to study carbon membrane. Compared to polymeric, carbon membranes possess high thermal and chemical resistance and higher pore volume with high separation factors [8].
Past few years, carbon membranes have attracted much attention as an alternative for polymeric membranes, owing to their good selectivity, high thermal strength, and high resistance against corrosion [4]. Basically, carbon membrane can be prepared by selected polymeric materials, and convert it to carbon membrane by carbonization process at controlled conditions [1]. There are two parameters that can be used to describe the performance of a membrane which are permeance and selectivity. Permeance of the membrane are the membrane’s processing capacity per unit time; which mean high productivity of the membrane produced from high permeance readings. Meanwhile, the selectivity of the membrane explains the membrane capacity to separate a desired component from the feed. High permeation flux and high selectivity are essential requirements for a successful membrane [2]. Carbon membranes are lack of being damaged in thermal cycles and easily handle at high temperatures when work with supported ceramic membrane.
Previous researchers stated that the biggest challenge in carbon membranes is their reproducible separation properties. Moreover, the carbon membranes prepared under different parameter will results in different separation performances [5]. However, carbon membranes have continuously earned world attention and considered as one of future nominee to replace any others membrane such as polymeric membranes. There are a lot of studies of carbon membranes such as membrane synthesis, characterization, membrane fabrication, and theoretical modelling have been done to get a superior membrane performance. As a result, the interest in carbon membrane application in various separation developments has been increased.
In general, carbon membranes can be grouped into two categories. Unsupported carbon membranes which is unsupported carbon membranes (flat, capillary or hollow fiber) [7] and supported carbon membranes (flat or tube [9]. Carbon tubular membranes has been utilized extensively in the carbon membranes preparation due to their high packing density and high separation performance from the stand point of large scale application. This approach can also overcome the brittleness of the prepared carbon membrane. The tubular supported carbon membranes are therefore regarded as the favoured choice for commercial application of carbon membranes owing to their thin separation layer and high mechanical strength. This paper aims to investigate the preparation of carbon membrane derived from Matrimid 5218 for CO2/CH4 separation.
2. Experimental
2.1. Materials
Matrimid® 5218 was purchased from Merck (Germany) and used as precursor material.N-methyl-2-pyrrolidone (NMP) was purchased from Sigma-Aldrich and used as a solvent. The commercial tubular ceramic (nominal cut-off 1kD, outer diameter: 13 mm, inner diameter: 11 mm, porosity: 40-50%, average pore size: 0.2 μm) was supplied by Shanghai Gongtao Ceramics Ltd. The membranes support with the length of 8 cm are made by TiO2 (Average particle size: 4.5-5.5 mm, Zinc oxide (ZrO2) (Average particle size: 2-3 nm) was coated on inner surface.
2.2. Carbon Membrane Preparation
Polymer solution was prepared by dissolving different Matrimid 5218 composition; 5wt%, 10wt%, 13wt%, 15 wt % and 18wt%, in N-methyl-2-pyrrolidone (NMP) for 7 hours with mechanical stirring. The mixing temperature used in this study is 80 oC. The dope comprising Matrimid 5218 and NMP was stirred around 24 hours to produce homogeneous solution. Finally the polymer solution is placed inside sonication bath in order to remove all existing bubbles for 24 hours. . In order to produce tubular membranes, ceramic tubes were coated with a mixture of 5-18 wt% Matrimid 5218 and NMP for 15 minutes on the outer surface of the support. Subsequently, the membrane was kept in a hygienic environment to let it curing for 24hours. Then, the membrane will be immersed in methanol for 2hours and put inside oven for drying purpose for 2hours. During the dip-coating method, the substrate is slowly dipped into and withdrawn from a tank containing the solution, with a uniform velocity, in order to obtain a uniform coating. Pinhole defects can lead to an unacceptable deterioration and sometimes loss of the usual separation ability of the resultant membranes. Thus, a three time’s dip-coating cycle was performed in this study in an attempt to avoid pinhole defects during polymer tubular membrane preparation. The heat treatment process has been done by placing the precursor membrane at the center of Carbolite (Model CTF 12/65/550) wire wound tube furnace. The length of the furnace tube is about 75 cm (heating zone 60 cm) and the diameter is 12 cm. A ceramic tube with the subsequent dimensions: 80 mm (length), 13 mm OD, and 10 mm internal diameter is located inside the furnace. Both ends of the ceramic tube were sealed with Pyrex glass cap. One of the ends is attached to the gas tank whereas the other end was open (purge stream). Before starting the heating cycle, the gas was allowable to flow throughout the furnace for several minutes. The polymeric membranes were heated at a heating rate of 2°C/min under Nitrogen gas with flow rate 200 ml/min. After completing each heating cycle, membranes were cooled naturally to room temperature. The detailed carbonization protocol is illustrated in Figure 1.
2.3. Membrane Characterizations
The characteristic of the gas penetrates, especially their kinetic diameter (refer Table 1), is necessary when considering the separation properties of the carbon membrane. This is because; carbon membrane is capable to separates gases by a molecular sieving mechanism. During the gas permeation test, the gas flow rate is dependent on the time taken for the gas to permeate at a constant volume of 1.0 ml. The gas pressure was varied 8 bars to determine the best pressure for the performance of the membranes. The feed pressure was regulated by the regulator on the gas cylinder and the needle valve. Permeate side exit from bore side of the tubular membrane were flowed straightforward to the bubble flow meter (burette) whereas the retentive (residue) gas was eliminated to the atmosphere. The permeance, P/l (GPU) and selectivity, α of the membranes were calculated using equations below:
Permeance, P:
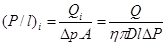
Selectivity, α:
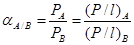
where P/l is the permeance of the membrane, Qi is the volumetric flow rate of gas i at standard temperature and pressure (cm3 (STP/s), p is the pressure difference between the feed side and the permeation side of the membrane (cmHg), A is the membrane surface area (cm2), n is the number of fibers in the module, D is an outer diameter of the membrane (cm) and l is an effective length of the membrane (cm).
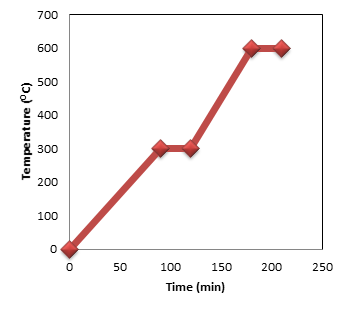
Table 1. Gas molecules characteristic.
Gas | Kinetic Diameter ( Å) |
N2 | 3.64 |
CO2 | 3.30 |
CH4 | 3.80 |
3. Results and Discussion
3.1. Membrane morphology
The carbonization process was performed by heating the Matrimid-based polymeric membrane under Nitrogen flow from room temperature to the final carbonization temperature. The permeance was measured using gas permeation test apparatus at 8 bars and room temperature. The results of the permeation performance of the prepared carbon membrane at different polymer composition are shown in Table 2. It was observed that the permeation of all gases increased with increasing polymer concentration to 15wt%. However, the permeance of all gases starts to decrease at polymer composition of 18wt %. This indicates that pore and carbon structure of the prepared carbon membrane become rigid, compact and some of the pores might change into closed pores during the carbonization process and hinder gas to permeate. It was observed that the prepared membrane become more selective as polymer concentration increase, from 5wt% to 15wt%.
Table 2. Gas Permeation Properties of the Matrimid –based Carbon Tubular Membrane.
Polymer concentration | Permeability (GPU) | Selectivity | |||
CO2 | CH4 | N2 | CO2/CH4 | CO2/N2 | |
5 wt% | 102.01±3.22 | 1.802±2.65 | 1.96±2.87 | 56.62±1.76 | 52.045±2.54 |
10 wt% | 115.9±4.21 | 1.872±1.76 | 2.11±3.49 | 61.93±2.25 | 54.9432±1.66 |
13 wt% | 137.9±1.98 | 1.929±2.44 | 2.163±1.17 | 71.45±1.64 | 63.723±3.41 |
15 wt% | 171.45±2.54 | 2.058±2.75 | 2.264±1.03 | 83.30±2.77 | 75.73±2.86 |
18 wt% | 145.7±2.19 | 1.973±1.41 | 2.175±2.52 | 73.86±3.68 | 67.00±1.92 |
As discussed earlier, higher polymer concentration leads to higher selectivity due to higher porosity. Since kinetic diameter of CO2 (3.3Å) and CH4 (3.8Å), the increase in CO2 permeance are more prominent. As a result, CO2/CH4 selectivity increases as polymer concentration increase to 15wt%. The selectivity of carbon membrane start to decrease at polymer concentration of 18wt% due to subtraction collapse and form unselective voids [3].
4. Conclusion
The carbon membrane prepared using different polymer precursor composition has been discussed and shows significant impact on gas separation properties. The different polymer composition gave different solvent exchange rate and resulted different carbon membrane morphology. Throughout the study, dip-coating carbon membrane derives from Matrimid® composition of 15wt% has produced the highest CO2 permeance CO2/CH4 selectivity of 83.30 with high gas pair selectivity. The work presented here has profound implications on future study of dip-coating carbon membrane and may one day help solve the problem of development of defect-free carbon membrane.
Acknowledgements
The authors gratefully acknowledge the financial support from Ministry of Higher Education and Universiti Teknologi Malaysia (UTM) under Higher Institution Centre of Excellence Scheme (Project Number : R.J090301.7846.4J188) and research University Grant Schemer (Project Number: Q. J130000.25546.12H76; Q.J130000.2642.10J79). The authors would also like to acknowledge technical and management support from Research Management Centre (RMC), Universiti Teknologi Malaysia.
References
[1] E. Barbosa-Coutinho, V. M. M. Salim and C. Piacsek Borges, "Preparation of carbon hollow fiber membranes by pyrolysis of polyetherimide," Carbon, vol. 41, pp. 1707-1714, 2003. View Article
[2] E. K. Chatzidaki, E. P. Favvas, S. K. Papageorgiou, N. K. Kanellopoulos and N. V. Theophilou, "New polyimide–polyaniline hollow fibers: Synthesis, characterization and behavior in gas separation," European Polymer Journal, vol. 43, pp. 5010-5016, 2007. View Article
[3] L.-H. Cheng, Y.-J. Fu, K.-S. Liao, J.-T. Chen, C.-C. Hu, W.-S. Hung, K.-R. Lee and J.-Y. Lai, "A high-permeance supported carbon molecular sieve membrane fabricated by plasma-enhanced chemical vapor deposition followed by carbonization for CO2 capture," Journal of Membrane Science, vol. 460, pp. 1-8, 2014. View Article
[4] A. Fernández-Barquín, C. Casado-Coterillo, M. Palomino, S. Valencia and A. Irabien, "Permselectivity improvement in membranes for CO2/N2 separation," Separation and Purification Technology, vol. 157, pp. 102-111, 2016. View Article
[5] X. He and M.-B. HäGg, "Optimization of Carbonization Process for Preparation of High Performance Hollow Fiber Carbon Membranes," Industrial & Engineering Chemistry Research, vol. 50, pp. 8065-8072, 2011. View Article
[6] Y. Li, C. Cao, T.-S. Chung and K. P. Pramoda, "Fabrication of dual-layer polyethersulfone (PES) hollow fiber membranes with an ultrathin dense-selective layer for gas separation," Journal of Membrane Science, vol. 245, pp. 53-60, 2004. View Article
[7] L. Liu, E. S. Sanders, S. S. Kulkarni, D. J. Hasse and W. J. Koros, "Sub-ambient temperature flue gas carbon dioxide capture via Matrimid® hollow fiber membranes," Journal of Membrane Science, vol. 465, pp. 49-55, 2014. View Article
[8] X. Ning and W. J. Koros, "Carbon molecular sieve membranes derived from Matrimid® polyimide for nitrogen/methane separation," Carbon, vol. 66, pp. 511-522, 2014. View Article
[9] C. Song, T. Wang, H. Jiang, X. Wang, Y. Cao and J. Qiu, "Gas separation performance of C/CMS membranes derived from poly(furfuryl alcohol) (PFA) with different chemical structure," Journal of Membrane Science, vol. 361, pp. 22-27, 2010. View Article
[10] W. N. Wan Salleh and A. F. Ismail, "Effect of stabilization temperature on gas permeation properties of carbon hollow fiber membrane," Journal of Applied Polymer Science, vol. 127, pp. 2840-2846, 2013. View Article
[11] B. Zornoza, C. Téllez and J. Coronas, "Mixed matrix membranes comprising glassy polymers and dispersed mesoporous silica spheres for gas separation," Journal of Membrane Science, vol. 368, pp. 100-109, 2011. View Article